Could it be that important interactions inside the cell or on the cell surface remain hidden from most measurements in the life sciences? This question has puzzled the team of laser and biophysicist Prof. Dr. Alexander Rohrbach from the Cluster of Excellence CIBSS at the University of Freiburg for years. He and his colleague Dr. Felix Jünger have been investigating various interactions between particles in the size range of bacteria, i.e. a few micrometres, and even of viruses, around 0.1 micrometres, on different cell surfaces. Using refined laser measurement technology and mathematical analysis methods, they have now succeeded in making previously hidden interactions visible. The results have been published in the journal Small. In the future, they may help to better understand how different particles bind to cells - be it viruses, bacteria, fine dust, cell debris or micelle-coated active ingredients.
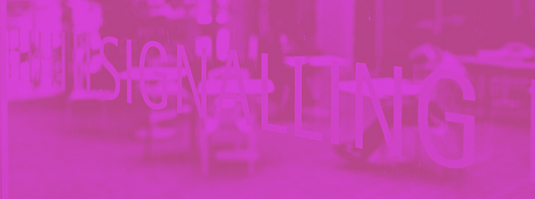
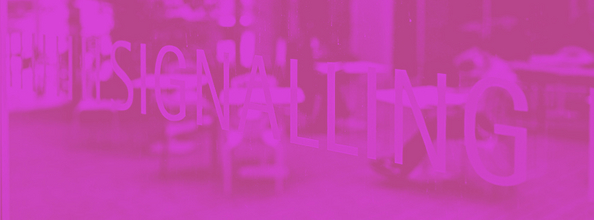
Hidden particle interactions at the cell surface revealed
Freiburg researchers find that ultra-smooth interactions can be detected through high-speed measurements and frequency analysis
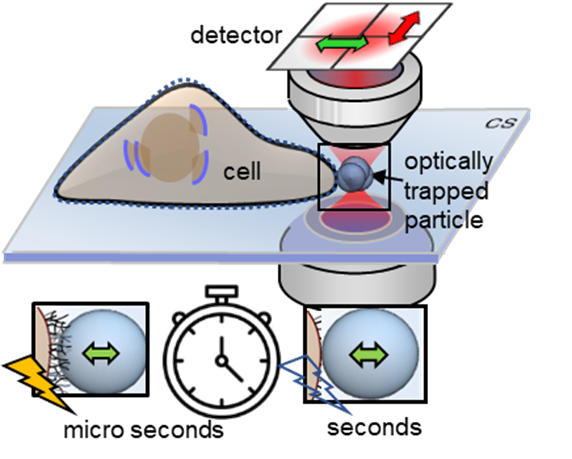
Microstructures, such as the glycocalyx on the cell surface, can be indirectly measured at the microsecond scale by fluctuating particles. Illustration: Alexander Rohrbach
Viscoelasticity determines behaviour
The viscoelastic properties of cell surfaces play a crucial role. Rohrbach gives the example of a solution of corn starch. "If you stir a sufficient amount of corn starch into a tub of water and walk on it quickly, you can actually walk on the liquid. All you feel is an elastic surface. But if you walk slowly or stop, you sink in and feel the liquid around your feet. So, depending on the time scale, the solution is either elastic or viscous. The viewer sees either a person with dry or wet feet stepping out of the tub.
Biological cells are made up of tiny molecular structures that can only be seen with the best microscopes. They all react to pressure or tension partly elastically, i.e. energy is stored, and partly viscously, i.e. energy is lost. Each individual cell is a viscoelastic system and thus determines the viscoelastic properties of, for example, muscle or connective tissue. Almost every cell has its own highly specialised extracellular matrix: a network of filamentous molecules, fine fibres and thin finger-like projections. This complex cell surface influences the uptake of particles such as viruses, bacteria or particles. "During signal transduction, the extracellular matrix not only distinguishes according to the size, shape and surface of the particles, but also according to how quickly or slowly thermally diffusing particles contact the surface, i.e. it acts as a spatial and temporal sieve for mechanical signals," explains Rohrbach.
Extremely fast measurement technology
Using a so-called photonic force microscope, a combination of optical laser tweezers and an interferometric 4-quadrant detection system, biophysicist Jünger first brought one-micrometer beads close to living cells in numerous experiments. The particle, which serves as a probe, is trapped in the laser focus but still makes small tremulous movements, known as thermal position fluctuations. These movements are measured in three dimensions a million times per second - with an accuracy of a few nanometres. However, the noisy position signals of the particle contain crucial information about the interaction with its environment.
If the particle is brought slowly to the cell surface with optical tweezers, or if the particle is presented to the cell at a short, constant distance, the interaction of the particle with the fine structures of the extracellular matrix begins within a few seconds. If you look at the position histogram, which is a distribution of all the particle positions, you will see that there is virtually no difference between the distribution before and after the interaction. The interaction is hidden. "The fast signal sampling allowed us for the first time to perform Kramers-Kronig integral transformations for thermal particle fluctuations, which allow us to visualise the elastic and viscous behaviour of cell structures at different motion frequencies," explains Jünger. Rohrbach adds: "However, to make sense of these two frequency responses, you have to put your idea of what's happening at the molecular level into mathematical equations and then compare how well the solutions to these equations match the experimental results."
Mathematical minimal model in frequency domain
The Freiburg researchers developed a minimal mathematical model in the frequency domain. This model can be adapted unexpectedly well to the viscoelastic behaviour in different measuring methods on different cells. By analysing the probe fluctuations, the scientists were able to determine important properties of the pericellular matrix (PCM) in intestinal epithelial cells, which consists of mesh-like hyaluronic acid strands. "We were able to measure the thickness of the PCM of 350 nanometres on the microsecond scale alone; on longer time scales, the PCM was simply invisible," says Rohrbach. The elasticity of the PCM, which is only about six pascals at millisecond dynamics, but about 20 pascals at microsecond dynamics, could explain, for example, why small and highly dynamic viruses tend to bounce off the PCM more elastically from a physical point of view, while larger and less dynamic bacteria tend to sink into the PCM, precisely because it is less elastic on longer time scales.
Original publication
Jünger, F., Rohrbach, A.: Making Hidden Cell Particle Interactions Visible by Thermal Noise Frequency Decomposition. In: Small (2023). DOI: 10.1002/smll.202207032